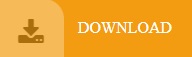
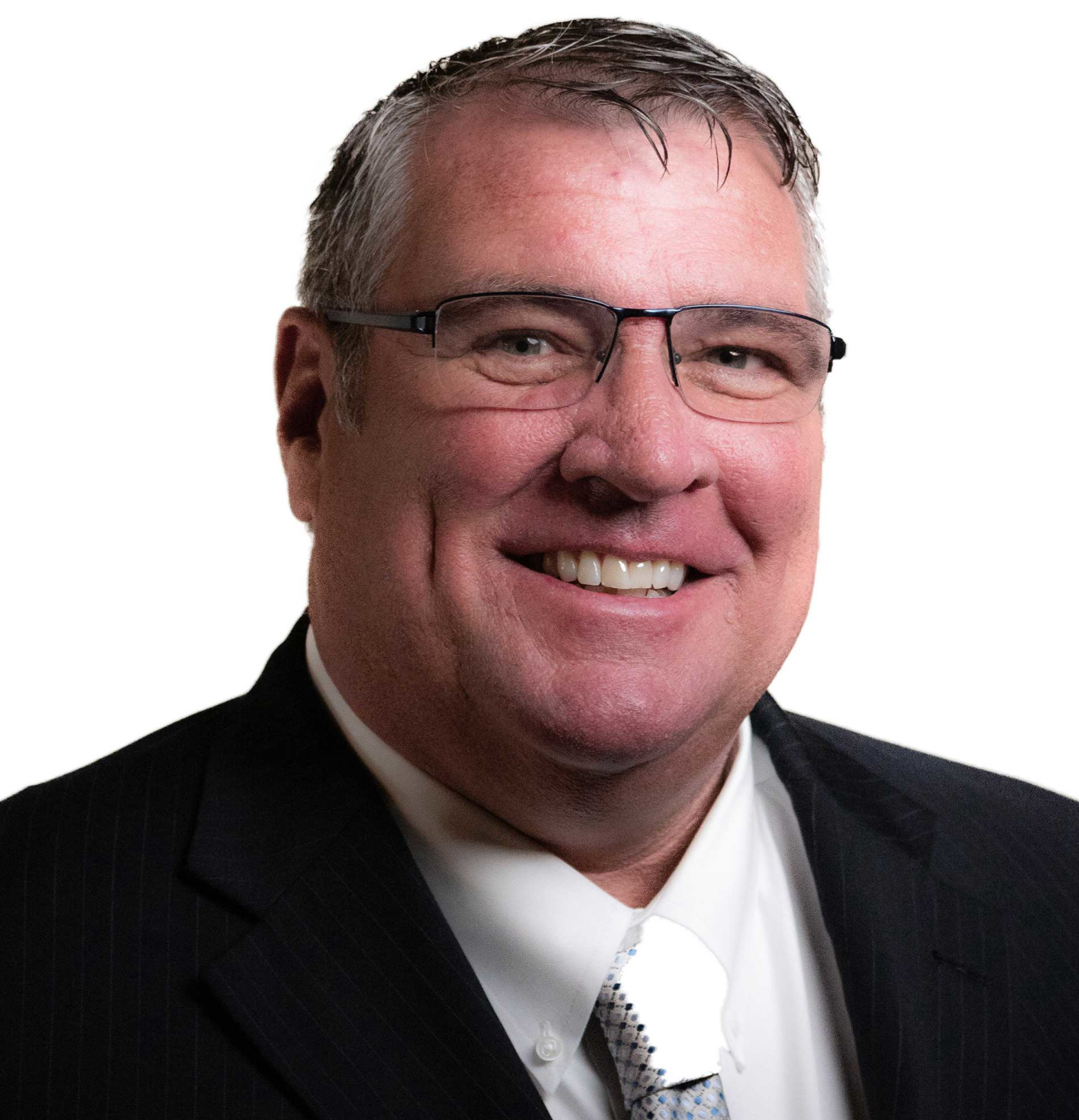
The support of this process by experimental techniques such as solid-state magic-angle spinning (MAS) NMR spectroscopy (14,15) can significantly reduce the complexity of the problem.įigure 6 shows a comparison of the asymmetric unit cell of the TFM LT (left, a and b) and TFM RT (right, c and d) polymorphs. (13) In such cases, the CSP search space grows exponentially and the prediction of experimentally observed crystal structures becomes ever more challenging. (12) Organic compounds are more challenging, since they tend to have a considerable conformational flexibility and can crystallize in a variety of conformations. (6,7) The ab initio random structure search (AIRSS) method that has been used successfully for a number of inorganic solids (8−11) has also been applied to a simple organic molecule. Several successful CSP studies on large but relatively rigid systems, such as organic porous cages, have been reported. The most stable (global minimum) structure provides a prediction of an experimental crystal structure. (1−5) CSP methods are based on searching for the most thermodynamically stable crystal structure, making various approximations in evaluating the crystal energy. In the group of theoretical methods, crystal structure prediction (CSP) developed during the last two decades has become one of the most promising approaches. For crystalline compounds, X-ray diffraction and solid-state NMR are usually the experimental techniques of choice.
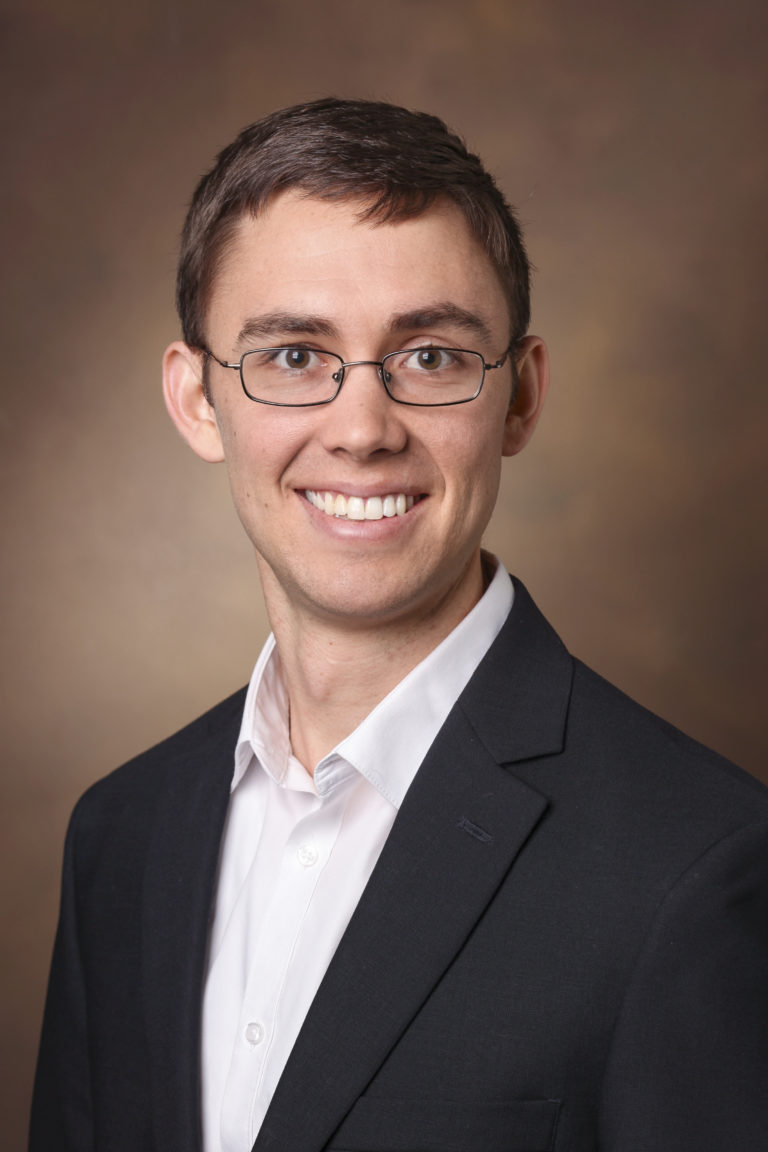
Understanding the complexity of such solid matter which depends on the interplay of a number of subtle, differentiated intermolecular contacts requires the application of different diagnostic tools and should be supported by theoretical methods. One particular scientific method is usually not enough to solve a challenging problem relating to solid matter for organic molecules, such as a moderately sized active pharmaceutical ingredient (API) organic molecule. Our study shows the power of combining experiments, namely DSC, X-ray diffraction, and MAS NMR, with DFT calculations and CSP to probe and understand the solid-state landscape, and in particular the role of dynamics, for pharmaceutical molecules. PISEMA solid-state NMR experiments show a reduction in the C–H dipolar coupling in comparison to the static limit for the aromatic CH moieties of 75% and 51% at 20 and 40 ☌, respectively, that is indicative of ring flips at the higher temperature. These observations are consistent with a broad energy minimum as revealed by DFT calculations. In the low-temperature structure, there are two torsion angles of 4.5 and 31.9° for the two Z’ = 2 molecules, while in the room-temperature structure, there is disorder that is modeled with ∼50% occupancy between torsion angles of −7.8 and 28.6°. Differences between the polymorphs are seen for the torsional angle describing the orientation of the phenyl ring relative to the planarity of the TFM molecule.

A crystal structure prediction procedure found both experimental teriflunomide polymorphs in the energetic global minimum region. Two-dimensional 13C– 1H and 1H– 1H double-quantum MAS NMR spectra are consistent with the new room-temperature structure, including comparison with GIPAW (gauge-including projector augmented waves) calculated NMR chemical shifts.

This reversible process is related to a change in Z’ value, from 2 to 1, as observed by variable-temperature 1H– 13C cross-polarization (CP) magic-angle spinning (MAS) solid-state NMR, while the crystallographic system is preserved (triclinic). undergoes a reversible thermal transition at −40 ☌. As revealed by DSC, the low-temperature TFM polymorph recently reported by Gunnam et al.
#Vanderbilt synergy code#
In this work, for the first time, we present the X-ray diffraction crystal structure and spectral properties of a new, room-temperature polymorph of teriflunomide (TFM), CSD code 1969989.
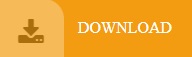